Diversity & Inclusion
The ATLAS Collaboration
The ATLAS Collaboration draws its creativity and strength from world-wide members with different backgrounds. This pluralism is an essential part of our identity as scientists and we fully uphold CERN's principles of inclusiveness and diversity as enshrined in the CERN Code of Conduct.
As a collaboration of over 5000 scientists, students, engineers, technicians, and administrators, ATLAS is made up of diverse members from around the globe with different age, gender identity, sexual orientation, culture, physical ability, race and ethnicity, appearance, neurodiversity, education, or religious background. We expect high standards of professional conduct and commitment to equality, diversity and inclusion in our community.
We value the opinions of people with different experiences and backgrounds. A diverse group brings different perspectives and enhances our ability to tackle complex problems. We expect colleagues to refrain from all types of bullying and harassment, including any form of abuse or exclusionary jokes at all times (e.g. racist, sexist) in person and in a virtual environment.
We recognise that discrimination persists in many aspects of society, including science. Racial harassment is unacceptable in all of its forms. We acknowledge that more must be done to address this. To be part of the solution, we continue to strive to identify and remove barriers that obstruct people from being able to work free from harassment, and to quickly address issues that may prevent everyone in the Collaboration from having equality of opportunity.
We must ensure that our colleagues have the tools and resources they need to succeed. Parenting and caring responsibilities can be challenging, traditionally having a higher impact on women, and we must ensure that support is in place. We must also take particular care to address the needs expressed by collaborators with various physical differences, such as vision, speech, hearing or other impairments.
We support LGBTQ+ rights. People are accepted for who they are, and we do not accept any discrimination due to sexual orientation or gender identity.
Science is about looking forward, and imagining a future that is inclusive, and celebrates diversity, ideas and innovation. Physics is universal and for everyone, and we must work to appreciate problems that others have that may be invisible to us.
This work is coordinated by the Contacts for Diversity and Inclusion, who also liaise with other groups including the other LHC experiments, the CERN Diversity and Inclusion Programme, and the CERN LGBTQ group.
ATLAS Colouring Book - Svenska (Swedish)
Design Guidelines
ATLAS Visual Identity
The ATLAS Collaboration has a professional visual identity that is both memorable and easy to recognise. It includes fonts, a colour palette and a refined logo, to help identify the ATLAS "brand". By embracing these design guidelines across all of our communication platforms, ATLAS presents a clear, professional image that all of our target audiences can identify.
ATLAS Collaboration members are encouraged to use this visual identity in talks and posters presented at conferences.
On this page you will find details and download that should help you in creating your talk, poster or other material.
ATLAS Logo
The official ATLAS logo plays a key role in establishing the Collaboration's visual identity. Ensure you use one of the variations available below.
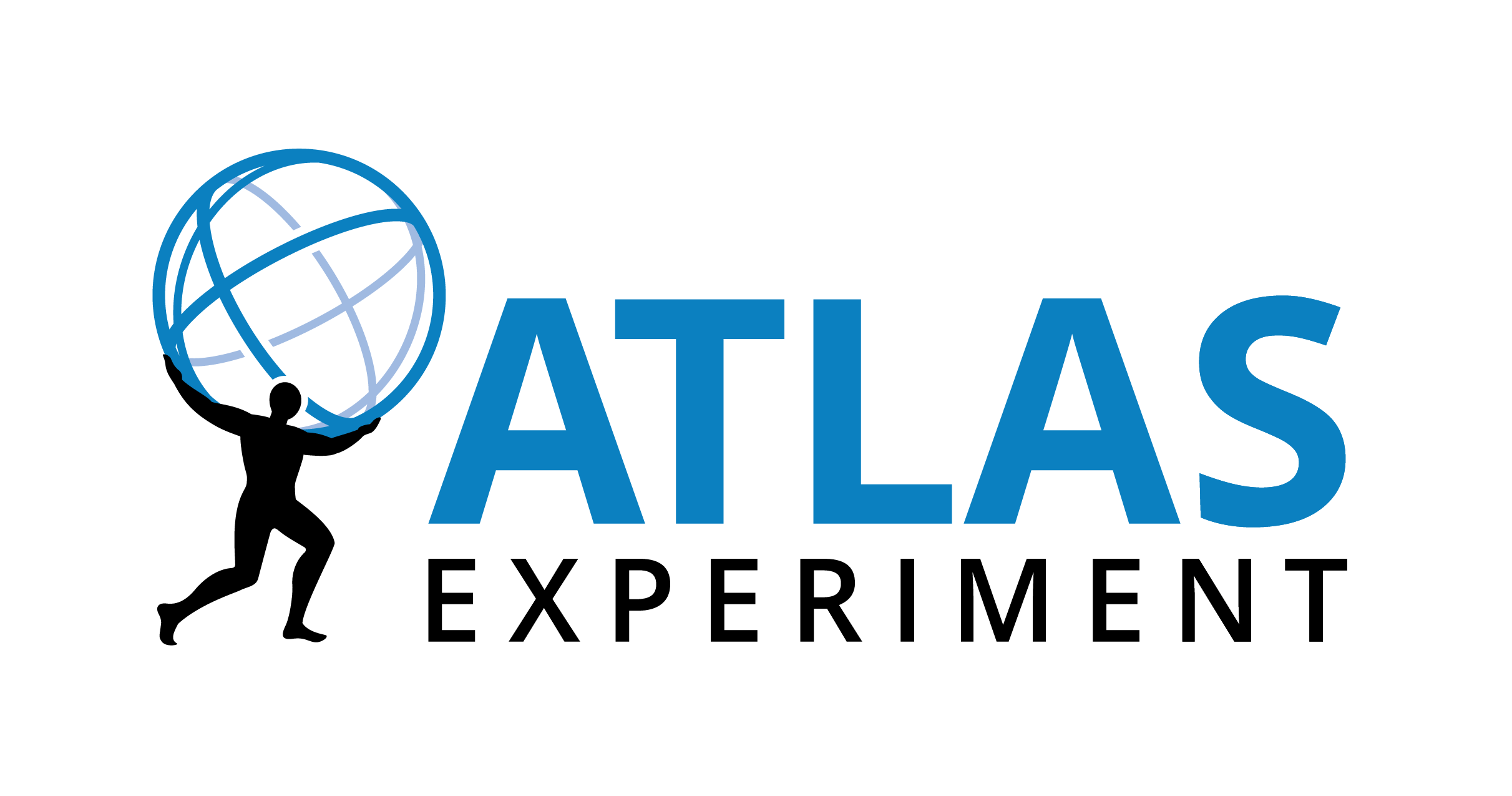
The standard ATLAS logo should be used on a clean white background. Otherwise, please use one of the approved logo variations below (click on them to get higher-resolution versions).
Members of the ATLAS Collaboration can download a full set of ATLAS logos in various formats (PNG, EPS, PDF, AI, ...) as well as animated versions of the logo from our CERNbox.
Run 3 Patch and Sticker Designs
Designs created by K. Anthony (patch, sticker) and H. Russell (sticker) in celebration of LHC Run 3. Members of the ATLAS Collaboration can download the pdf files of the designs from CERNbox.
Fueled by ML/AI Design
Design created by E. Prado for use by the ATLAS Collaboration. Members of the ATLAS Collaboration can download the pdf/ai files of the designs from CERNbox.
ATLAS Colours
The ATLAS website and visual identity uses a standard "ATLAS blue" colour.
HEX: #0b80c3
RGB: 11, 128, 195
CMYK: 83, 42, 1, 0
ATLAS Font
The default ATLAS font is Open Sans, and is used in the ATLAS logo, website and official communications. Open Sans is an open source font available for free in the Google Fonts database.
Presentation and Poster Templates
ATLAS Collaboration members can download ATLAS presentation themes/templates for Keynote and Powerpoint, as well as a PowerPoint poster template from our CERNbox share.
Guidelines for ATLAS members
Acknowledgements and proper crediting of ATLAS contribution by third parties and partners is done through copyright for images, and comments for written content.
Using the ATLAS logo on a web site or any document is totally different: given that it is a brand, the logo implies that the collaboration directly contributed to or endorsed the project through an established partnership where roles and contributions have been clearly defined, and that the material was checked in details.
Projects which require the right to use the ATLAS brand therefore agree to go through approval, reporting and to implement the modifications required. In this situation, the role of the outreach coordinators is to interact with the project developers, advise and collect background information until the material has reached a sufficient quality to be presented to the relevant bodies. Approval is then given (or not) by the ATLAS management.
The right to use the ATLAS logo and brand can be removed at any time. In that case the project is of course not stopped, the logo and mention of ATLAS are removed and it runs with other partners. For this reason – and to simplify Intellectual Property issues - it is strongly recommended to chose a neutral project name.
Note that the use of the CERN logo requires a similar approval by the CERN communication and legal services, and that the use of the letters CERN in project names is generally not accepted.
Terms of use
Particles of the Universe Colouring Book - English
The Higgs boson
A landmark discovery
What is the Higgs boson and why does it matter?
Physicists describe particle interactions using the mathematics of field theory, in which forces are carried by intermediate particles called bosons. Photons, for example, are bosons carrying the electromagnetic force. In 1964, the only mathematically consistent theory required bosons to be massless. Yet, experiment showed that the carriers of the weak nuclear interaction – the W and Z bosons – had large masses. To solve this problem, three teams of theorists: Robert Brout and François Englert; Peter Higgs; Gerald Guralnik, Carl Hagen, and Tom Kibble independently proposed a solution now referred to as the Brout-Englert-Higgs (BEH) mechanism.
The BEH mechanism required the presence of a new field throughout the universe which gave mass to some of the bosons. Existence of this field could be verified by discovery of its associated particle – the Higgs boson. On 4 July 2012, the ATLAS and CMS experiments at CERN announced that they had independently observed a new particle in the mass region of around 125 GeV: a boson consistent with the Higgs boson. On 8 October 2013, the Nobel Prize in Physics was awarded jointly to theorists François Englert and Peter Higgs "for the theoretical discovery of a mechanism that contributes to our understanding of the origin of mass of subatomic particles, and which recently was confirmed through the discovery of the predicted fundamental particle, by the ATLAS and CMS experiments at CERN's Large Hadron Collider".
The discovery of the Higgs boson opened up whole new windows in the search for new physics, since its properties are predicted to be different in different theoretical models. Supersymmetry, for example, predicts the existence of at least five different types of Higgs bosons. Will the Standard Model continue to survive the precision measurements of the LHC or will an improved model appear? Only through continued study will physicists be able to answer this question.
What have we learned since discovery?
After discovery, physicists began to study the properties of the newly-discovered particle to understand if it was the Standard Model Higgs boson or something else. First came the confirmation of the mass of the Higgs boson: the final unknown parameter in the Standard Model. This was one of the first parameters measured and found to be approximately 125 GeV (roughly 130 times larger than the mass of the proton). With this mass, the Higgs boson could decay several different ways.
In the Standard Model, the Higgs boson is unique: it has zero spin, no electric charge and no strong force interaction. The spin and parity were measured through angular correlations between the particles it decayed to. Sure enough, these properties were found to be as predicted. At this point, physicists began to call it “the Higgs boson.” Of course, it still remains to be seen if it is the only Higgs boson or one of many, such as those predicted by supersymmetry.
Subsequent studies by ATLAS and CMS have found that the Higgs boson interacts with both bosons and fermions (particles that make up matter), confirming the prediction by the Standard Model that all elementary particles acquire mass via the all-pervasive Higgs field. The stronger a particle interacts with the Higgs field, the heavier is. Physicists have also been studying the strength of these interactions, which could be impacted by new physics beyond the Standard Model.
The first direct probe of fermionic interactions was to tau particles, which was observed in the combination of ATLAS and CMS results performed at the end of Run 1. During Run 2, the increase in the centre-of-mass energy to 13 TeV and the larger dataset allowed further channels to be probed. Further, the Higgs boson has been observed decaying to bottom quarks and being produced together with top quarks. This means that the interaction of the Higgs boson to fermions has been clearly established.
One of the neatest ways to summarise the current understanding of the Higgs boson is by comparing its interaction strength to the mass of Standard Model particles (see figure on left). This clearly shows that the interaction strength depends on the particle mass: the heavier the particle, the stronger its interaction with the Higgs field. This is one of the main predictions of the BEH mechanism in the Standard Model.
Physicists are not only trying to verify that the properties of the Higgs boson agree with those predicted by the Standard Model – they are specifically looking at what properties would provide evidence for new physics! For example, constraining the rate that the Higgs boson decays to invisible or unobserved particles provides stringent limits on the existence of new particles with masses below that of the Higgs boson. They are also looking for Higgs boson decays to combinations of particles forbidden in the Standard Model. So far, none of these searches have found anything unexpected, but the search is still on!
Highlights of ATLAS' exploration of the Higgs boson
The Higgs boson
A landmark discovery
What is the Higgs boson and why does it matter?
Physicists describe particle interactions using the mathematics of quantum field theory, in which forces are carried by intermediate particles called bosons. Photons, for example, are bosons carrying the electromagnetic force. In 1964, the only mathematically consistent theory required bosons to be massless. Yet, experiments showed that the carriers of the weak nuclear interaction – the W and Z bosons – had large masses. To solve this problem, three teams of theorists: Robert Brout and François Englert; Peter Higgs; Gerald Guralnik, Carl Hagen, and Tom Kibble independently proposed a solution now referred to as the Brout-Englert-Higgs (BEH) mechanism.
The BEH mechanism requires the presence of a new field throughout the universe which gives mass to some of the bosons. Existence of this field could be verified by discovery of its associated particle – the Higgs boson. On 4 July 2012, the ATLAS and CMS experiments at CERN announced that they had independently observed a new particle in the mass region of around 125 GeV: a particle consistent with the Higgs boson. On 8 October 2013, the Nobel Prize in Physics was awarded jointly to theorists François Englert and Peter Higgs "for the theoretical discovery of a mechanism that contributes to our understanding of the origin of mass of subatomic particles, and which recently was confirmed through the discovery of the predicted fundamental particle, by the ATLAS and CMS experiments at CERN's Large Hadron Collider".
The discovery of the Higgs boson has opened up new windows in the search for new physics phenomena, since its properties – and even the number of distinct types of Higgs boson – are predicted to be different in different theoretical models. Supersymmetry, for example, predicts the existence of at least five different types of Higgs bosons. Many theories also predict that the Higgs boson plays a critical role in the production of new phenomena such as dark matter at the LHC. Higgs bosons now become a tool for physicists, studying the properties of particles produced alongside the Higgs boson in search of new discoveries.
Will the Standard Model continue to survive the precision measurements of the LHC or will an improved model appear? Only through continued study and more data will physicists be able to answer this question.
What have we learned since discovery?
Immediately after the discovery, physicists began to study the properties of the newly-found particle to understand if it was the Standard Model Higgs boson or something else. First came the confirmation of the mass of the Higgs boson: the final unknown parameter in the Standard Model. This was one of the first parameters measured and found to be approximately 125 GeV (roughly 130 times larger than the mass of the proton). With this mass, the Higgs boson could decay in several different ways.
The discovery of the Higgs boson opened a whole new branch of particle physics, as researchers began to study its properties. In the Standard Model, the Higgs boson is unique: it has zero spin (making it the only fundamental particle with this characteristic), no electric charge and no strong force interaction. The spin and parity were measured through angular correlations between the particles it decayed to. Sure enough, these properties were found to be as predicted. At this point, physicists began to call it “the Higgs boson.” It still remains to be seen if it is the only Higgs boson or one of many, such as those predicted by supersymmetry. Some theories predicting extra Higgs bosons have already been ruled out, but other theories will require more data to explore their prediction.
By studying the production and decay of the Higgs boson, ATLAS physicists confirmed that the Higgs boson interacts with both bosons and fermions (the latter being particles that make up matter), confirming the prediction by the Standard Model that elementary particles acquire mass via the all-pervasive Higgs field.
The discovery of the Higgs boson relied on measurements of its decay to vector bosons: the photon, W and Z. The existence of such interactions provided crucial evidence for a process known as spontaneous electroweak symmetry breaking to have occurred in our Universe, and is a key prediction of the theory of the BEH mechanism. However, measurements of this kind were not enough to give the complete picture of the Higgs boson’s properties. In the Standard Model, different types of couplings determine the Higgs boson’s interactions with fermions and bosons, so the presence of new undiscovered physics might impact them differently.
The first direct probe of fermionic interactions was the decay to tau particles, which was observed in the combination of ATLAS and CMS results performed at the end of Run 1. During Run 2, the increase in the centre-of-mass energy to 13 TeV and the larger dataset allowed further channels to be probed. Subsequently, the Higgs boson was observed decaying to bottom quarks and being produced together with top quarks. More recently, the first measurements have been made of Higgs boson couplings to second-generation fermions, such as the charm quark and the muon. The interaction of the Higgs boson with matter particles has now been clearly established.
One of the most illustrative ways to summarise the current understanding of the Higgs boson’s couplings to other particles is by comparing its interaction strength to the mass of Standard Model particles (see figure on left). This clearly shows that the interaction strength (vertical axis) depends on the particle mass (horizontal axis): the heavier the particle, the stronger its interaction with the Higgs field. This is one of the main predictions of the BEH mechanism in the Standard Model.
Can the Higgs boson be used as a tool for future discovery?
The relation between the Higgs boson’s coupling strength and particle mass is not the only property of its interactions that physicists want to understand. Larger datasets collected since the original discovery, and data still to be recorded, now enable detailed and precise measurements of how often the Higgs boson is produced and decays through various different mechanisms.
The figure on right illustrates just some of the measurements of the production and decay rates of the Higgs boson (vertical axes) that have been made. Any deviations from the predictions of the Standard Model could indicate our understanding of the Higgs boson is incomplete.
By looking at the motion of the particles from the decay of the Higgs boson, such as the angles between the particles produced, other properties of Higgs boson interactions can be tested. Such techniques have been used to explore whether Higgs interactions with tau particles exhibit charge-parity asymmetry and thus whether the Higgs boson plays a special role in explaining why there is vastly more matter than antimatter in our Universe.
One as-of-yet unobserved interaction predicted for the Higgs boson is its self-interaction, where Higgs bosons interact with each other. This is an extremely rare process at the LHC and is predicted to require much more data than currently recorded to even be observed. This process allows exploration of new phenomena predicted to only interact with the Higgs boson, but also crucially will allow tests of properties of the Higgs field itself for the first time.
Physicists are not just trying to verify that the properties of the Higgs boson agree (or not) with those predicted by the Standard Model – they are also now using the Higgs boson as a tool to search for evidence for new physics!
With measurement of the Higgs boson to known particles firmly established, a comprehensive search programme is underway using what we know about the Higgs boson to study theories predicting new particles produced from the decay of a Higgs boson, or produced alongside it.
Measurements are being made testing theories which predict Higgs boson decays to combinations of particles forbidden in the Standard Model or setting stringent constraints on theories that predict new particles to be produced in the decay of the Higgs boson (see figure here).
A particular puzzle the Higgs boson is being used to probe is the mystery of dark matter. As dark matter has mass, the BEH mechanism tells us the Higgs boson should interact with it. Physicists use this knowledge to both search for dark matter produced alongside Higgs bosons in the LHC and, by constraining the rate that the Higgs boson decays to invisible or unobserved particles, search for dark matter produced in from Higgs bosons themselves. These searches put strong constraints on theories of dark matter interactions with normal matter (see figure on left). So far, none of these searches have found anything unexpected, but the search is still on and there is still much to explore!
Highlights of ATLAS' exploration of the Higgs boson
Resources
ATLAS on Flickr
Image | Anyone
Tags: photos, gifs, images
ATLAS on YouTube
Video | Anyone
Tags: videos, animations
ATLAS Schematics
Image | Anyone
Tags: Schematics, videos, images, animations
ATLAS Posters
Poster | Anyone
Tags: education, outreach, open days, public engagement
Seasonal Activities
Activity | Anyone
Tags: education, outreach, Stencils, activities
ATLAS 3D Printed Model
Activity | Primary Students, Secondary Students, University Students, Anyone
Tags: detector
ATLAS Open Data
Activity | Secondary Students, University Students, Citizen Scientists
Tags: open data
ATLAS@Home
Activity | Citizen Scientists
Tags: citizen science
Fact Sheets
Brochure | Secondary Students, University Students, Teachers, Anyone
Tags: fact sheets, printables
External Resources for Citizen Scientists
Links | Citizen Scientists
Tags: games, activities
Resources
ATLAS on Flickr
Image | Anyone
Tags: photos, gifs, images
ATLAS on YouTube
Video | Anyone
Tags: videos, animations
ATLAS Schematics
Image | Anyone
Tags: Schematics, videos, images, animations
ATLAS Posters
Poster | Anyone
Tags: education, outreach, open days, public engagement
ATLAS Colouring Books
Book | Primary Students, Teachers
Tags: standard model, detector, collaboration
Seasonal Activities
Activity | Anyone
Tags: education, outreach, Stencils, activities
ATLAS 3D Printed Model
Activity | Primary Students, Secondary Students, University Students, Anyone
Tags: detector
ATLAS Virtual Visits
Activity | Secondary Students, Teachers
Tags: virtual visits, classroom
ATLAScraft
Game | Primary Students, Secondary Students, Teachers
Tags: multiplayer, detector
International Physics Masterclasses
Activity | Teachers
Tags: international masterclasses
Cheat Sheets
Brochure | Secondary Students, University Students, Teachers
Tags: fact sheets, printables
Fact Sheets
Brochure | Secondary Students, University Students, Teachers, Anyone
Tags: fact sheets, printables
External Resources for Teachers
Links | Teachers
Tags: games, activities
Resources
ATLAS on Flickr
Image | Anyone
Tags: photos, gifs, images
ATLAS on YouTube
Video | Anyone
Tags: videos, animations
ATLAS Schematics
Image | Anyone
Tags: Schematics, videos, images, animations
ATLAS Posters
Poster | Anyone
Tags: education, outreach, open days, public engagement
Seasonal Activities
Activity | Anyone
Tags: education, outreach, Stencils, activities
ATLAS 3D Printed Model
Activity | Primary Students, Secondary Students, University Students, Anyone
Tags: detector
ATLAS PhD Grant
Activity | University Students
Tags: ATLAS PhD grant
ATLAS Open Data
Activity | Secondary Students, University Students, Citizen Scientists
Tags: open data
Cheat Sheets
Brochure | Secondary Students, University Students, Teachers
Tags: fact sheets, printables
Fact Sheets
Brochure | Secondary Students, University Students, Teachers, Anyone
Tags: fact sheets, printables
External Resources for University Students
Links | University Students
Tags: games, activities