Join as a Technical Associate Institute
The status of a Technical Associate Institute is designed for research laboratories or universities that seek to have a close cooperation with the ATLAS Collaboration on primarily technical work for a dedicated project over an extended (multi-year) but limited period of time. They do not intend to join the ATLAS Collaboration as a full member Institution and do not intend to contribute to the ATLAS physics programme.
The principal reason for a Technical Associate membership is cooperation on one or more challenging technical project(s) relevant for the ATLAS Collaboration. These projects are possible in the areas of detector development, engineering, electronics, firmware, software and computing. They may be linked to detector upgrade programmes. Engagement in R&D activities that could potentially be of interest for ATLAS is possible as well. There should be clear benefit to the group joining, as well as to ATLAS, from the application.
Given that no full membership is anticipated, no financial contribution to enter the collaboration and no M&O costs have to be paid. Technical Associate Institutes are not required to contribute to the OT tasks; however, they are welcome to do so, concerning the maintenance and operation of their deliverables.
Technical Associate Institutes appear under a separate heading on the list of ATLAS Institutions. While members from the Technical Associated Institutes do not sign the physics publications of the ATLAS Collaboration, they sign all project-related technical papers where they are involved together with authors from the ATLAS Collaboration.
Path to Technical Associate Institute membership
- Initial contact should be with the ATLAS Spokesperson or with Project or Activity Leaders of Technical Projects in ATLAS.
- The applicant group should prepare an Expression of Interest detailing the interest of the group in a specific project, its background and the involved personnel (scientist, engineers and students) as well as the timeline of the common project between the ATLAS Collaboration and the applicant group and, linked to this, the duration of the association.
- The applicant group will be associated to one or more ATLAS detector systems and/or activity areas and well-defined deliverables should be defined. Long-term maintenance and OT linked to the deliverable of a Technical Associate Institute is the responsibility of the respective system or activity area. The Project Leaders and/or Activity Coordinators have to find appropriate groups (ATLAS Institutions) to cover this. Therefore, it is desirable that Technical Associate Institutes collaborate closely with existing ATLAS Institutions. It is also possible that the Technical Associate Institutes stay engaged beyond the development and construction phase and take longer-term commitments for operation.
- Technical Associate Institutes might partake in the resources available for technical projects in ATLAS, e.g. for upgrade projects or for software and computing projects, from central ATLAS resources or from resources in the respective countries. In the latter case the agreement of the respective national ATLAS community and the funding agency is required and a supporting letter from the corresponding NCP is expected.
- Before Technical Associate Institute status is granted, an agreement between the Technical Associate Institute and CERN (as the Host Laboratory) has to be prepared. Such an agreement should specify the details and deliverables of the project as well as ownership and definition of responsibilities for maintenance and operations. Where the technical project involves a contribution to an ATLAS Upgrade Project, the Technical Associate Institute has to comply with the agreed ATLAS procedures for preparation and implementation including critical reviews and timelines.
- The agreement with CERN is presented by the Spokesperson to the ATLAS CB, and a formal vote on the admission is taken. After admission by the CB and after signature of the agreement the Technical Associate Institute status is granted. The admission of a new Technical Associate Institute does not require an endorsement by the RRB, as the CB composition is unchanged; however, the RRB would be notified.
Interested in ATLAS membership?
Join as an associate institute
The Associate Institute status is intended for universities or research labs that are on the path to joining ATLAS as a full institutional member.
The Associate Institute is hosted by a current ATLAS full-member Institution, which takes some responsibility for the associated group. Members of the Associate Institute will collaborate with the host Institution. There should be clear benefit shown to the Institution being joined, as well as to ATLAS, from the application.
Associate Institutes do not appear on the list of Institutions in the ATLAS author list. Scientific authors from Associate Institutes instead appear as authors of the host Institution, with an “Also at [Associate Institute]” footnote upon request. Associate Institutes are generally not a visible part of the ATLAS Collaboration. They do not appear in the CERN "Grey Book", nor do they have the right to open a Team Account at CERN based on their ATLAS Associate Institute status.
Path to Associate Institute membership
- Initial contact should be made with the team leader of the Institution proposed, or directly with the ATLAS Spokesperson, who will put the group in touch with nearby member Institutions, if appropriate. In any case, informal contact should be made at an early stage with the Spokesperson and with the NCP of the nation concerned.
- The applicant group should prepare a short statement of interest, including a description of the general structure (staff, students, engineers) and background of the group, expectations for its evolution, specific interests (projects, activity areas in ATLAS) and work programme. It is expected that members of the applicant institute will begin qualification to become ATLAS authors.
- Statements of support will be required from the team leader of the Institution being joined and the NCP, who should also ensure that the funding agency concerned is in agreement.
- With an Associate Institute joining, the institutional responsibilities (M&O and OT shares) are increased. These are assigned to the host Institution, which should agree with the Associate Institute how they will be met. Payment of the additional M&O contributions will start for the calendar year after admission.
- The acceptance of an Associate Institute is at the discretion of the Spokesperson and Collaboration Board chair. The Collaboration Board shall be informed and comments solicited, before acceptance is implemented. The admission of a new Associate Institute does not require a CB vote or endorsement by the RRB, as the CB composition is unchanged.
Interested in ATLAS membership?
JOIN AS A CLUSTERED INSTITUTION
Clustered Institutions (Clusters) are typically intended for institutes in nations where the High-Energy Physics community is still developing, and where it may be difficult to form individual university groups large enough to stand alone in ATLAS. It is expected that members of a Cluster (Cluster institutes) work closely together and build up a coherent effort in ATLAS.
Each Cluster will have one vote in the ATLAS Collaboration Board.
The requirements for a new Cluster to join ATLAS are the same as for single Institutions. The obligations on joining fees, M&O and OT contributions have to be fulfilled by the Cluster as a whole.
The requirements for a new institute joining a Cluster as an additional institute are less stringent. No entrance fee has to be paid, however the financial and effort obligations (M&O and OT contributions) of the Cluster increase according to the number of additional authors. The application should benefit the Cluster being joined, as well as the ATLAS Collaboration as a whole.
Clusters appear – and are marked as such – in the list of institutes in ATLAS publications. The individual Cluster institutes are listed consecutively.
Path to Clustered Institution membership
- Initial contact should be made with the leader of the Cluster team the new institute proposes to join, or directly with the ATLAS Spokesperson. In any case, informal contact should be made at an early stage with the Spokesperson and with the National Contact Physicist (NCP) of the nation concerned.
- The applicant group should prepare a short statement of interest, including a description of the general structure (staff, students, engineers) and background of the group, expectations for its evolution, specific interests (projects, activity areas in ATLAS) and work programme. Connections and relations to other institutes in the Cluster they wish to join should be described. Members of the applicant institute are expected to begin qualification to become ATLAS authors.
- Support will be required from the leader of the Cluster being joined and the NCP of the nation, who should also ensure that the funding agency concerned is in agreement.
- The applicant institute, and the Cluster they propose to join, need to accept the financial and effort implications of joining, i.e. increased shares of M&O and OT contributions, commensurate with the number of additional authors, qualifiers and students (see above). Payment of the additional M&O contributions will start for the calendar year after admission. The leader of the Cluster is responsible for ensuring that these commitments will be met by the engaged institutes in the Cluster.
- The addition of the institute to the Cluster is at the discretion of the Spokesperson and Collaboration Board chair. The Collaboration Board shall be informed and comments solicited, before the admission to the Cluster is completed. The admission of a new institute into a Cluster does not require a CB vote or endorsement by the RRB, as the CB composition is unchanged.
Interested in ATLAS membership?
Join as an Institution
Full Institutional Members (Institutions) have full rights and obligations in the Collaboration. In particular, they are represented in the Collaboration Board (CB). This is an assembly of all ATLAS Institutions, where major decisions for the collaboration are discussed and voted on. Every Institution is equal, with one vote each.
ATLAS Institutions are expected to contribute to the Operation and Maintenance (M&O), to Operation Tasks (OT), and physics programme of the ATLAS experiment, as well as to the detector upgrade programme for operation at the High-Luminosity LHC. ATLAS Institutions have the following obligations:
- To enter the ATLAS Collaboration as a full Institutional Member, a financial contribution must be paid. This contribution can, in part, be delivered “in-kind”, by providing well-defined deliverables in technical areas, e.g. software or firmware. Details are to be discussed with the Spokesperson.
- There is a yearly share of M&O costs of around 10 kCHF per author (or qualifying author) holding a PhD or equivalent (M&O author). In addition, a common fund contribution of about 1.5 kCHF per M&O author, per year is paid during the High-Luminosity LHC detector upgrade phase (2018–2025). There are no M&O payments for students.
- Institutions take on a share of the OT, such as shifts or data quality monitoring, and corresponding institutional commitments. For the OT requirements students are also included, however with a lower weight of 0.75. An increased OT share is expected to be taken by the joining groups during the first two years.
- New groups are also expected to contribute to the Phase-II upgrade of the ATLAS experiment.
- The admission of a new full member Institution is taken by a vote at the CB and endorsement by the Resources Review Board (RRB) need to be obtained.
Path to Institutional membership
- A new group usually starts working with ATLAS before any formal engagement is taken. This happens most efficiently by being hosted by an existing ATLAS Institution, e.g. as an Associate Institute (see below). This initial work helps to establish and foster the mutual interest for future long-term Institutional Membership.
- After about one year, the new group may submit an Expression of Interest (EoI) to join the ATLAS Collaboration, describing its general structure (staff, students, engineers), the expertise of the group, its planned activities and contributions to ATLAS as well as its projected evolution. At that stage the group must demonstrate a critical size, i.e. it should have permanent staff and typically more than one faculty member. The group must have a well-defined set of interests and a plan for long-term engagement within the collaboration.
- The EoI is announced by the Spokesperson to the ATLAS CB, followed by a short presentation of the group leader of the applicant institute. Earliest one CB later (there are three per year) a formal vote on the admission can be taken. A statement of support is required from the National Contact Physicist of the respective nation, who should also ensure that both the ATLAS national community (if it exists already) and the funding agency/ies concerned are in agreement. In addition, a supporting statement from the Project Leader or Activity Coordinator of the areas where the new group plans to get engaged is required.
Interested in ATLAS membership?
Collaboration membership
ATLAS Membership
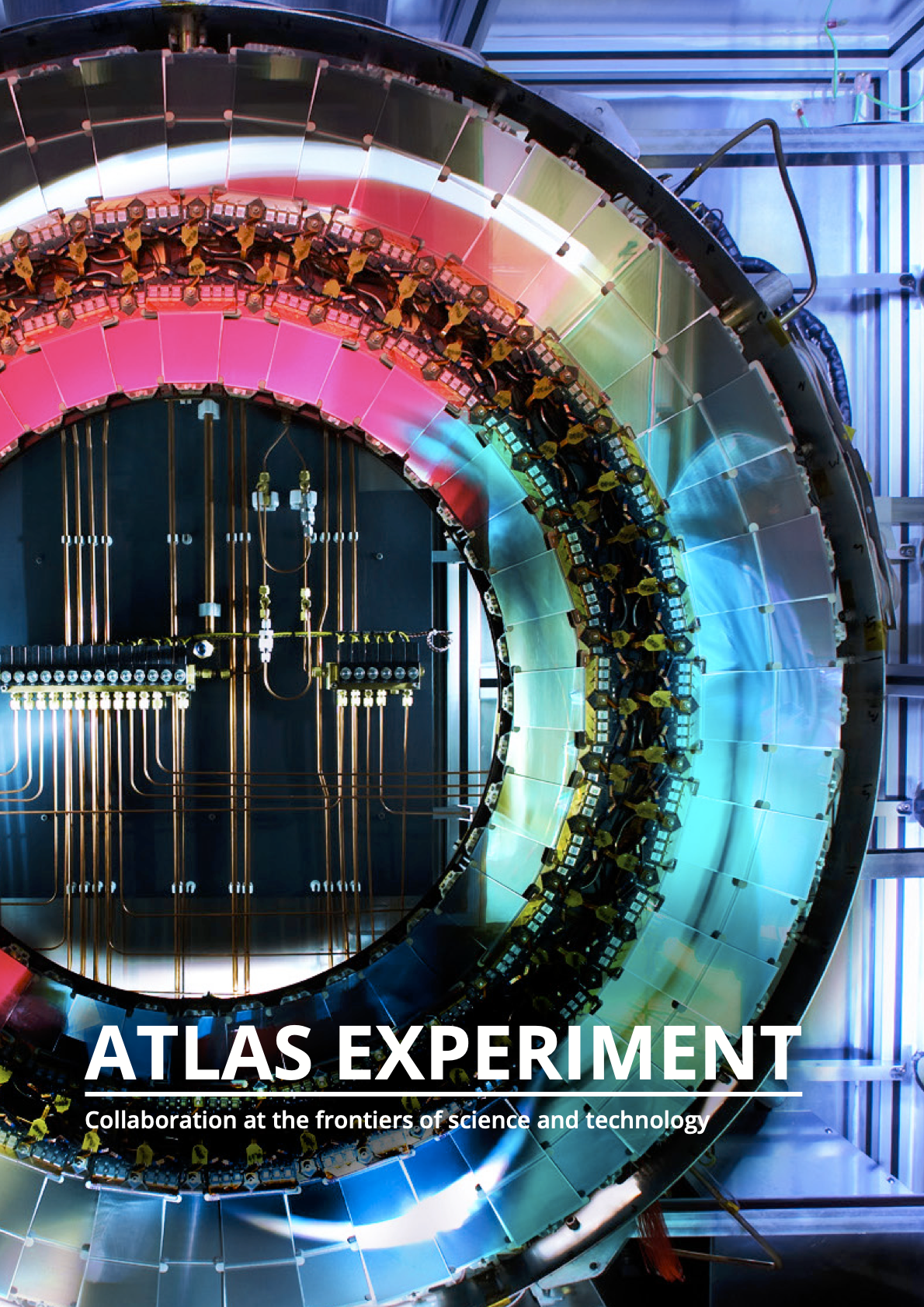
Institutes joining the ATLAS Collaboration participate in the great endeavour of exploring the smallest constituents of matter and the nature of the fundamental forces acting between them. The high energies and high intensities provided by the LHC allow for the exploration of new territory in the search for new particles and in the study of rare processes. New results on these frontiers might also advance the understanding of the history of our universe.
The unprecedented experimental challenges faced by ATLAS require the advancement of technology in electronics, computing and data processing. Work is carried out by a global collaboration of scientists, engineers and technicians. The stimulating international environment of ATLAS and CERN, combined with work on the frontline of physics and technology, form an exciting scientific endeavour that offers a unique training ground for students and young researchers.
Types of Membership
There are several types of institutional membership in the ATLAS Collaboration. Full Institutional Membership can either be obtained by single institutes or by several smaller institutes that work together and join the ATLAS Collaboration as a Clustered Institution. In addition, institutes can be associated to a full member Institution as an Associate Institute. Institutes interested in a cooperation with the ATLAS Collaboration on technical projects can be affiliated as Technical Associate Institutes.
Join as an Institution
Full Institutional Members (Institutions) have full rights and obligations in the Collaboration. In particular, they are represented in the Collaboration Board (CB). This is an assembly of all ATLAS Institutions, where major decisions for the collaboration are discussed and voted on. Every Institution is equal, with one vote each.
ATLAS Institutions are expected to contribute to the Operation and Maintenance (M&O), to Operation Tasks (OT), and physics programme of the ATLAS experiment, as well as to the detector upgrade programme for operation at the High-Luminosity LHC. ATLAS Institutions have the following obligations:
To enter the ATLAS Collaboration as a full Institutional Member, a financial contribution must be paid. This contribution can, in part, be delivered “in-kind”, by providing well-defined deliverables in technical areas, e.g. software or firmware. Details are to be discussed with the Spokesperson.
There is a yearly share of M&O costs of around 10 kCHF per author (or qualifying author) holding a PhD or equivalent (M&O author). In addition, a common fund contribution of about 1.5 kCHF per M&O author, per year is paid during the High-Luminosity LHC detector upgrade phase (2018–2025). There are no M&O payments for students.
Institutions take on a share of the OT, such as shifts or data quality monitoring, and corresponding institutional commitments. For the OT requirements students are also included, however with a lower weight of 0.75. An increased OT share is expected to be taken by the joining groups during the first two years.
New groups are also expected to contribute to the Phase-II upgrade of the ATLAS experiment.
The admission of a new full member Institution is taken by a vote at the CB and endorsement by the Resources Review Board (RRB) need to be obtained.
Path to Institutional Membership
- A new group usually starts working with ATLAS before any formal engagement is taken. This happens most efficiently by being hosted by an existing ATLAS Institution, e.g. as an Associate Institute (see below). This initial work helps to establish and foster the mutual interest for future long-term Institutional Membership.
- After about one year, the new group may submit an Expression of Interest (EoI) to join the ATLAS Collaboration, describing its general structure (staff, students, engineers), the expertise of the group, its planned activities and contributions to ATLAS as well as its projected evolution. At that stage the group must demonstrate a critical size, i.e. it should have permanent staff and typically more than one faculty member. The group must have a well-defined set of interests and a plan for long-term engagement within the collaboration.
- The EoI is announced by the Spokesperson to the ATLAS CB, followed by a short presentation of the group leader of the applicant institute. Earliest one CB later (there are three per year) a formal vote on the admission can be taken. A statement of support is required from the National Contact Physicist of the respective nation, who should also ensure that both the ATLAS national community (if it exists already) and the funding agency/ies concerned are in agreement. In addition, a supporting statement from the Project Leader or Activity Coordinator of the areas where the new group plans to get engaged is required.
Join as a Clustered Institution
Clustered Institutions (Clusters) are typically intended for institutes in nations where the High-Energy Physics community is still developing, and where it may be difficult to form individual university groups large enough to stand alone in ATLAS. It is expected that members of a Cluster (Cluster institutes) work closely together and build up a coherent effort in ATLAS.
Each Cluster will have one vote in the ATLAS Collaboration Board.
The requirements for a new Cluster to join ATLAS are the same as for single Institutions. The obligations on joining fees, M&O and OT contributions have to be fulfilled by the Cluster as a whole.
The requirements for a new institute joining a Cluster as an additional institute are less stringent. No entrance fee has to be paid, however the financial and effort obligations (M&O and OT contributions) of the Cluster increase according to the number of additional authors. The application should benefit the Cluster being joined, as well as the ATLAS Collaboration as a whole.
Clusters appear – and are marked as such – in the list of institutes in ATLAS publications. The individual Cluster institutes are listed consecutively.
Path to Clustered Institution membership
- Initial contact should be made with the leader of the Cluster team the new institute proposes to join, or directly with the ATLAS Spokesperson. In any case, informal contact should be made at an early stage with the Spokesperson and with the National Contact Physicist (NCP) of the nation concerned.
- The applicant group should prepare a short statement of interest, including a description of the general structure (staff, students, engineers) and background of the group, expectations for its evolution, specific interests (projects, activity areas in ATLAS) and work programme. Connections and relations to other institutes in the Cluster they wish to join should be described. Members of the applicant institute are expected to begin qualification to become ATLAS authors.
- Support will be required from the leader of the Cluster being joined and the NCP of the nation, who should also ensure that the funding agency concerned is in agreement.
- The applicant institute, and the Cluster they propose to join, need to accept the financial and effort implications of joining, i.e. increased shares of M&O and OT contributions, commensurate with the number of additional authors, qualifiers and students (see above). Payment of the additional M&O contributions will start for the calendar year after admission. The leader of the Cluster is responsible for ensuring that these commitments will be met by the engaged institutes in the Cluster.
- The addition of the institute to the Cluster is at the discretion of the Spokesperson and Collaboration Board chair. The Collaboration Board shall be informed and comments solicited, before the admission to the Cluster is completed. The admission of a new institute into a Cluster does not require a CB vote or endorsement by the RRB, as the CB composition is unchanged.
Join as an Associate Institute
The Associate Institute status is intended for universities or research labs that are on the path to joining ATLAS as a full institutional member.
The Associate Institute is hosted by a current ATLAS full-member Institution, which takes some responsibility for the associated group. Members of the Associate Institute will collaborate with the host Institution. There should be clear benefit shown to the Institution being joined, as well as to ATLAS, from the application.
Associate Institutes do not appear on the list of Institutions in the ATLAS author list. Scientific authors from Associate Institutes instead appear as authors of the host Institution, with an “Also at [Associate Institute]” footnote upon request.
Associate Institutes are generally not a visible part of the ATLAS Collaboration. They do not appear in the CERN "Grey Book", nor do they have the right to open a Team Account at CERN based on their ATLAS Associate Institute status.
Path to Associate Institute membership
- Initial contact should be made with the team leader of the Institution proposed, or directly with the ATLAS Spokesperson, who will put the group in touch with nearby member Institutions, if appropriate. In any case, informal contact should be made at an early stage with the Spokesperson and with the NCP of the nation concerned.
- The applicant group should prepare a short statement of interest, including a description of the general structure (staff, students, engineers) and background of the group, expectations for its evolution, specific interests (projects, activity areas in ATLAS) and work programme. It is expected that members of the applicant institute will begin qualification to become ATLAS authors.
- Statements of support will be required from the team leader of the Institution being joined and the NCP, who should also ensure that the funding agency concerned is in agreement.
- With an Associate Institute joining, the institutional responsibilities (M&O and OT shares) are increased. These are assigned to the host Institution, which should agree with the Associate Institute how they will be met. Payment of the additional M&O contributions will start for the calendar year after admission.
- The acceptance of an Associate Institute is at the discretion of the Spokesperson and Collaboration Board chair. The Collaboration Board shall be informed and comments solicited, before acceptance is implemented. The admission of a new Associate Institute does not require a CB vote or endorsement by the RRB, as the CB composition is unchanged.
Join as a Technical Associate Institute
The status of a Technical Associate Institute is designed for research laboratories or universities that seek to have a close cooperation with the ATLAS Collaboration on primarily technical work for a dedicated project over an extended (multi-year) but limited period of time. They do not intend to join the ATLAS Collaboration as a full member Institution and do not intend to contribute to the ATLAS physics programme.
The principal reason for a Technical Associate membership is cooperation on one or more challenging technical project(s) relevant for the ATLAS Collaboration. These projects are possible in the areas of detector development, engineering, electronics, firmware, software and computing. They may be linked to detector upgrade programmes. Engagement in R&D activities that could potentially be of interest for ATLAS is possible as well. There should be clear benefit to the group joining, as well as to ATLAS, from the application.
Given that no full membership is anticipated, no financial contribution to enter the collaboration and no M&O costs have to be paid. Technical Associate Institutes are not required to contribute to the OT tasks; however, they are welcome to do so, concerning the maintenance and operation of their deliverables.
Technical Associate Institutes appear under a separate heading on the list of ATLAS Institutions. While members from the Technical Associated Institutes do not sign the physics publications of the ATLAS Collaboration, they sign all project-related technical papers where they are involved together with authors from the ATLAS Collaboration.
Path to Technical Associate Institute membership
- Initial contact should be with the ATLAS Spokesperson or with Project or Activity Leaders of Technical Projects in ATLAS.
- The applicant group should prepare an Expression of Interest detailing the interest of the group in a specific project, its background and the involved personnel (scientist, engineers and students) as well as the timeline of the common project between the ATLAS Collaboration and the applicant group and, linked to this, the duration of the association.
- The applicant group will be associated to one or more ATLAS detector systems and/or activity areas and well-defined deliverables should be defined. Long-term maintenance and OT linked to the deliverable of a Technical Associate Institute is the responsibility of the respective system or activity area. The Project Leaders and/or Activity Coordinators have to find appropriate groups (ATLAS Institutions) to cover this. Therefore, it is desirable that Technical Associate Institutes collaborate closely with existing ATLAS Institutions. It is also possible that the Technical Associate Institutes stay engaged beyond the development and construction phase and take longer-term commitments for operation.
- Technical Associate Institutes might partake in the resources available for technical projects in ATLAS, e.g. for upgrade projects or for software and computing projects, from central ATLAS resources or from resources in the respective countries. In the latter case the agreement of the respective national ATLAS community and the funding agency is required and a supporting letter from the corresponding NCP is expected.
- Before Technical Associate Institute status is granted, an agreement between the Technical Associate Institute and CERN (as the Host Laboratory) has to be prepared. Such an agreement should specify the details and deliverables of the project as well as ownership and definition of responsibilities for maintenance and operations. Where the technical project involves a contribution to an ATLAS Upgrade Project, the Technical Associate Institute has to comply with the agreed ATLAS procedures for preparation and implementation including critical reviews and timelines.
- The agreement with CERN is presented by the Spokesperson to the ATLAS CB, and a formal vote on the admission is taken. After admission by the CB and after signature of the agreement the Technical Associate Institute status is granted. The admission of a new Technical Associate Institute does not require an endorsement by the RRB, as the CB composition is unchanged; however, the RRB would be notified.
Interested in ATLAS membership?
The ATLAS Management
Current ATLAS Management
The Spokesperson and Deputy Spokespersons oversee all aspects of the ATLAS project. The Spokesperson represents ATLAS to CERN, funding agencies, and other external bodies. The Technical Coordinator oversees the construction and technical integration of ATLAS components, ensuring engineering standards and procedures are followed. The Resources Coordinator handles resource planning and administration of the ATLAS common fund, ensuring ATLAS resource needs are consistent with local national plans. The Upgrade Coordinator oversees the ATLAS upgrade programme, ensuring it is coherent, technically sound, and aligned with ATLAS physics goals.
Andreas Hoecker
Spokesperson 2021-2025, Deputy 2017-2021
Manuella Vincter
Deputy Spokesperson 2019-2025
Stéphane Willocq
Deputy Spokesperson 2023-2025
David Francis
Resource Coordinator 2019-2025
Martin Aleksa
Technical Coordinator 2024-2027
Benedetto Gorini
Upgrade Coordinator 2022-2025
Previous ATLAS Management
Ludovico Pontecorvo
Technical Coordinator 2015-2024
Marumi Kado
Deputy Spokesperson 2021-2023
Francesco Lanni
Upgrade Coordinator 2019-2022
Karl Jakobs
Spokesperson 2017-2021
Isabelle Wingerter-Seez
Deputy Spokesperson 2017-2019
Fido Dittus
Resource Coordinator 2013-2019
Kevin Einsweiler
Upgrade Coordinator 2017-2019
David Charlton
Spokesperson 2013-2017, Deputy 2009-2013
Beate Heinemann
Deputy Spokesperson 2013-2017
Robert McPherson
Deputy Spokesperson 2015-2017
Thorsten Wengler
Deputy Spokesperson 2013-2015
Beniamino Di Girolamo
Technical Coordinator 2013-2015
Fabiola Gianotti
Spokesperson 2009-2013, Deputy 2004-2009
Andy Lankford
Deputy Spokesperson 2009-2013
Markus Nordberg
Resource Coordinator 2001-2013
Marzio Nessi
Technical Coordinator 2001-2013
Peter Jenni
Spokesperson 1995-2009, Co-Spokesperson 1992-1995
Steinar Stapnes
Deputy Spokesperson 2004-2009
Torsten Åkesson
Deputy Spokesperson 1996-2004
Peter Schmid
Resource Coordinator 1994-2001
Michael Price
Technical Coordinator 1999-2001
Hans Hoffman
Technical Coordinator 1994-1999
Friedrich Dydak
Co-Spokesperson 1992-1995
The Collaboration
ATLAS is one of the largest collaborative efforts ever attempted in science.
Organisational Structure
ATLAS is a collaboration of physicists, engineers, technicians, students and support staff from around the world. It is one of the largest collaborative efforts ever attempted in science, with approximately 6000 members and 3000 scientific authors. The success of ATLAS relies on the close collaboration of research teams located at CERN, and at member universities and laboratories worldwide.
ATLAS elects its leadership and has an organisational structure that allows teams to self-manage, and members to be directly involved in decision-making processes. Scientists usually work in small groups, choosing the research areas and data that interest them most. Any output from the collaboration is shared by all members and is subject to rigorous review and fact-checking processes before results are made public. The success of the collaboration is bound by individual commitment to physics and the prospect of exciting new results that can only be achieved with a complete and coherent collaborative effort.
The only way to realise such a challenging project, with the required intellectual and financial resources, and to maximise its scientific output is through international collaboration. Large project funds are investments from funding agencies of countries participating in ATLAS. There are also contributions from CERN, and some resources from individual universities.
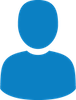
3000
Scientific authors
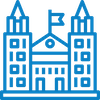
182
Institutions
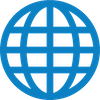
42
Countries
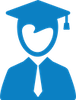
1200
Doctoral students
Caption: ATLAS member countries are shaded blue. Click on country for summary information (double-click to zoom). Orange markers indicate ATLAS institutes (click for information, double-click for zoom). Use mouse wheel or buttons to zoom (click on ocean or non-member area to jump back to world view). See the full list of ATLAS institutions here.
ATLAS Management
The ATLAS management team is responsible for overseeing all the aspects of the collaboration. It is led by a Spokesperson, who has the overall responsibility of the day-to-day organisation of ATLAS. Supporting the Spokesperson is one or two Deputy Spokespersons, whose responsibilities are defined by the Spokesperson. The team also includes a Technical Coordinator, who monitors the overall technical aspects of the experiment; a Resource Coordinator, responsible for the financial and human resources of the collaboration; and an Upgrade Coordinator, responsible for overseeing and monitoring the ATLAS upgrades. The ATLAS Collaboration Board elects its Spokesperson every two years and the position is renewable once (two election terms).
The Physics
ATLAS explores a range of physics topics, with the primary focus of improving our understanding of the fundamental constituents of matter.
What are the basic building blocks of matter?
The Standard Model describes the elementary subatomic particles of the universe which have been experimentally seen. ATLAS studies these particles and searches for others to determine if the particles we know are indeed elementary or if they are in fact composed of other more fundamental ones.
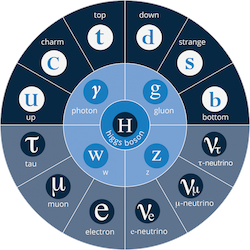
What are the forces that govern their interactions?
The Standard Model also describes the fundamental forces of Nature and how they act between fundamental particles. Possible discoveries at the LHC could validate models, such as those incorporating Supersymmetry, where the forces unify at very high energies.
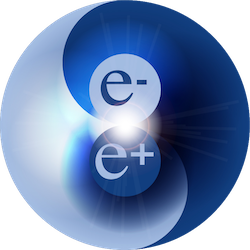
What happened to antimatter?
By searching for imbalances in the production of matter and antimatter, we seek to understand why our universe appears to comprise only matter.
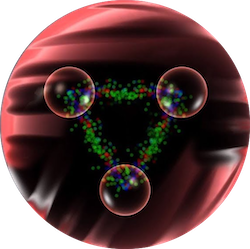
What is “dark matter”?
Astronomical measurements support the existence of matter that cannot be directly seen. The hermetic construction of ATLAS, however, makes it possible to search for this “dark matter”.
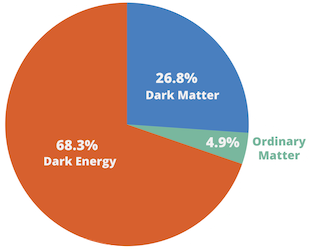
What was the early universe like and how will it evolve?
Proton-proton and heavy-ion collisions delivered by the LHC recreate the conditions immediately following the Big Bang when the Universe was governed by high-energy particle physics and later by a primordial soup of quarks and gluons, and allow ATLAS to study fundamental questions such as the Brout-Englert-Higgs field or Dark Matter.
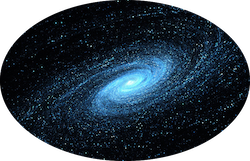
How does gravity fit in?
Gravity is extremely weak when compared to the other forces. To explain the difference we look for such exotic phenomena as extra dimensions, gravitons, and microscopic black holes.
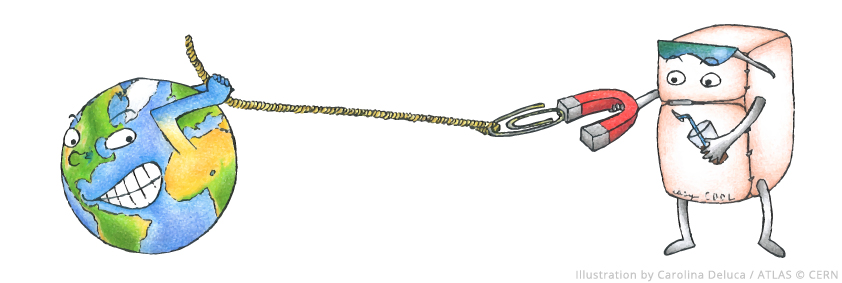
Anything else?
Perhaps the most exciting aspect of the ATLAS physics programme is our ability to explore and discover new phenomena beyond existing theoretical predictions: the search for the unknown.
The Higgs boson
What is the Higgs boson and why does it matter?
Physicists describe particle interactions using the mathematics of field theory, in which forces are carried by intermediate particles called bosons. Photons, for example, are bosons carrying the electromagnetic force. In 1964, the only mathematically consistent theory required bosons to be massless. Yet, experiment showed that the carriers of the weak nuclear interaction – the W and Z bosons – had large masses. To solve this problem, three teams of theorists: Robert Brout and François Englert; Peter Higgs; Gerald Guralnik, Carl Hagen, and Tom Kibble independently proposed a solution now referred to as the Brout-Englert-Higgs (BEH) mechanism.
The ATLAS Experiment
Push the frontiers of knowledge
ATLAS is a general-purpose particle physics experiment at the Large Hadron Collider (LHC) at CERN. It is designed to exploit the full discovery potential of the LHC, pushing the frontiers of scientific knowledge. ATLAS' exploration uses precision measurement to push the frontiers of knowledge by seeking answers to fundamental questions such as: What are the basic building blocks of matter? What are the fundamental forces of nature? What is dark matter made of?
Global Collaboration
ATLAS is a collaboration of physicists, engineers, technicians, students and support staff from around the world. It is one of the largest collaborative efforts ever attempted in science, with over 5500 members and almost 3000 scientific authors. The success of ATLAS relies on the close collaboration of research teams located at CERN, and at member universities and laboratories worldwide.
Experimental behemoth
ATLAS is the largest detector ever constructed for a particle collider: 46 metres long and 25 metres in diameter. Its construction pushed the limits of existing technology. ATLAS is designed to record the high-energy particle collisions of the LHC, which take place at a rate of over a billion interactions per second in the centre of the detector. More than 100 million sensitive electronics channels are used to record the particles produced by the collisions, which are then analysed by ATLAS scientists.
Understanding the Universe
ATLAS physicists are studying the fundamental constituents of matter to better understand the rules behind their interactions. Their research has lead to ground-breaking discoveries, such as that of the Higgs boson. The years ahead will be exciting as ATLAS takes experimental physics into unexplored territories – searching for new processes and particles that could change our understanding of energy and matter.
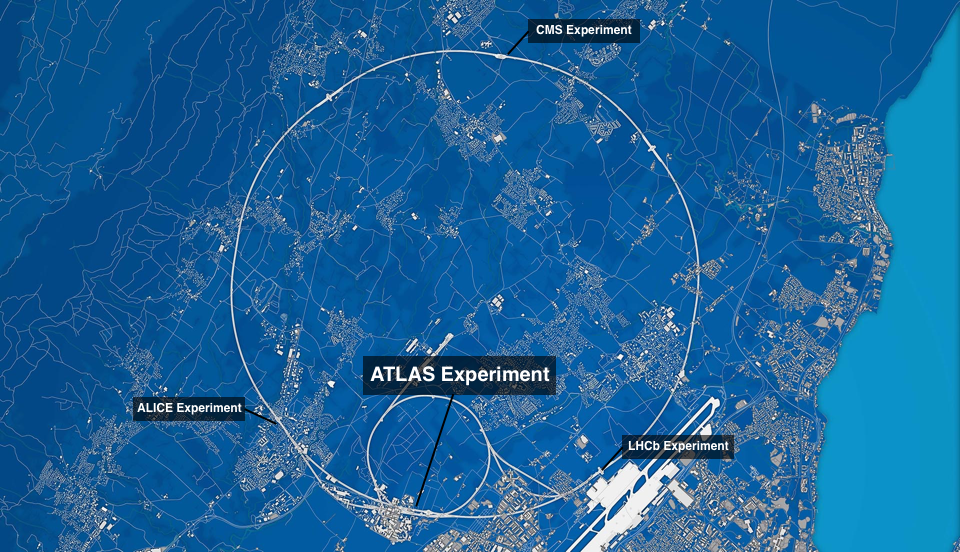
ATLAS Across Time
The approval of the ATLAS Experiment was an important milestone in the history of particle physics – but it was just the first step in a long journey. Making ATLAS a reality required years of innovative developments in technology and physics. Learn the history of its development in the timeline below.